The core raw material for multilayer ceramic capacitor (MLCC) is mainlyaconsists of barium titanate based ferroelectrics upon ferroelectric polarization effect. Since there ferroelectric phase lie near room temperature, the temperature/thermal stability of dielectric constant is relatively poor. Therefore, exploring a new giant dielectric material, excellent in temperature and frequency stability has become a hot spot in recent years, and has been regarded as breakthrough for novel MLCC electronic components. Acceptor-donor ambipolar ion doped metal oxides are promising candidates to show high-performance colossal permittivity (>1000) behavior based on electron-pinned defect-dipoles, which has drawn an increasing attention from many domestic and foreign scholars. Recently, the research team of Professor Qiuyun Fu and Associat Professor Wen Dong published an article "High-performance colossal permittivity behaviour persists to ultralow temperature in Co+Ta co-doped SnO2: A spin-defect mediated superstable large electronic moment of defect-dipole" in materials based top journal “ACTA MATERIALIA” where an unconventional thermo-activated dielectric relaxation behavior with defect-dipolar freezing temperature below 2k is reported for the first time. For convenience, Co-only doped SnO2, Co+Ta co-doped SnO2and Co+Nb co-doped SnO2is abbreviated as CSO,xCTSO, andxCTSO, wherexis the co-doping level.

Figure 1Mapping of theTffor defect-induced colossal permittivity in major host simple binary metal oxides (normal TADR region) in comparison with the CTSO (red star) (abnormal TADR region). The arrow indicate theTfis below 2 K and undetermined. (TADR: thermally activated dielectric relaxation).
The mechanism study of this non-intrinsic polarization induced colossal permittivity behavior is still in its primary stage, and most of the research focuses on the dielectric behavior shown in various doping systems. Different doping systems exhibit different colossal permittivity behavior and freezing temperature of defect-dipolesTf(as shown in Figure 1), which mainly corresponds to different electron staples. There is an urgent need to carry out more in-depth research to understand the electron-pinned defect-dipole effect and regulation methods. Most of the research systems are currently doped with the simple metal oxides using the host elements. The electron pinning effect manifested as trapping of electrons by energy wells, is mainly due to the role of Coulomb force. Based on these phenomena, the research team of Prof. Qiuyun Fu and Associate Prof. Wen Dong additionally introduces electron spin-coupling, that is, the spin correlation between local electronic spin and nearly free-charge (from donor) carrier spin.
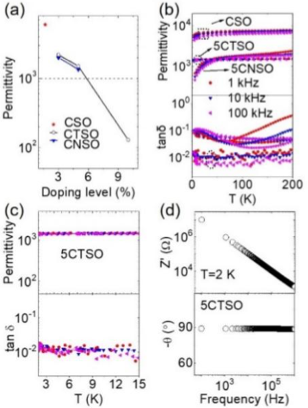
Figure 2 Structure and dielectric characterisation.(a) Dielectric permittivity of CSO, CNSO, and CTSO samples measured at 300 K and 1 MHz. (b) Temperature dependent dielectric spectra of CSO, 5CNSO, and 5CTSO samples from 2 K to 200 K. (c) The dielectric spectra of 5CTSO magnified in the temperature range of 2 K to 15 K. (d) Real impedance and phase angle as a function of frequency.
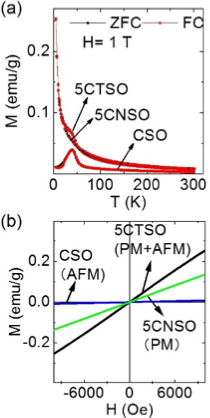
Figure 3 Magnetic properties.(a) Magnetization as a function of temperature for CSO, 5CNSO, and 5CTSO in zero-field-cooling (ZFC) and field-cooling (FC) process. The applied magnetic field is 1 T. (b) Magnetization as a function of applied magnetic field (-1 T to 1 T) for CSO, 5CNSO, and 5CTSO.
SnO2is selected as a parent material, as a giant dielectric transition temperature of the SnO2doping system has been reported elsewhere, providing/provides a large temperature window for the study of electronic pinning effect. Co2+is magnetic acceptor, Nb5+and Ta5+are selected as donor, respectively. As shown in Figure 2, Co single doping and Co + Nb, Co + Ta co-doped SnO2exhibited a giant dielectric behavior, wherein the giant dielectric transition temperature of the first two is 25 K and 7 K, respectively. While, unprecedently a stable colossal permittivity below 2K is observed without a giant dielectric transition behavior, indicating that the gauge electrical transition temperature is below 2 K. This is the first time to observe this critical electron-pinning effect in such materials. As shown in Figure 3, the Co-only doped SnO2is an antiferromagnetic, while the Co + Nb co-doped SnO2becomes magnetic, and the Co + Ta co-doped SnO2is a combination of antiferromagnetic and paramagnetic states, which is explained by the different magnetic coupling effect of Co+Nb and Co+Ta, where the former one has stronger magnetic coupling than later one.

Figure 4 Spin-defect mediated critical partial localization state.(a-c) Calculated local defect configurations of CSO, CTSO, and CNSO, respectively. The defect configurations of CSO and CTSO also include a spin density where the blue and yellow clouds around the Co atom indicate spin down (Co1) and spin up (Co2), respectively. Oxygen vacancyVOis indicated by the circular round dot. The DOS of the CSO and CTSO along with the partial DOS of Co1 are provided. (d) Freezing temperatureTfas a function of spin-defect mediated composition with the illustration of the critical state. It indicates that the magnetic states change from Co-Co coupling determined AFM state to a Co-M(M= Ta, Nb) coupling determined AFM+PM critical state (Tf< 2K) and further to Co-Nb coupling determined PM state. (e) The evolution of energy barrier by the spin-defect mediation along with rough illustrations of free energy landscape.
As shown in Figure 4, the theoretical calculations show that Co-only doped and Co+ Nb, Co + Ta co-doped SnO2shows different defect-dipole state. This is consistent with the results of the magnetic behaviors, and also corresponds to the critical electron coupling effect. The electron-critical magnetic state of the Co + Ta co-doped SnO2exhibits a special electron spin coupling effect, in which the very low temperature of thermo-activation of the pinned-electrons suggests a flattened free energy well. This is why the system exhibits an unconventional dielectric relaxation behavior very well below 2k.
The research outcomes provide a significant guidance for further development of defect induced giant capacitive materials and new MLCC devices, and the excellent dielectric behavior of Co+Ta co-doped SnO2is expected to be applied to ultra-low temperature electronic capacitive components.
The results are in cooperation/collaboration with University of Warwick, which has been financially supported by the National Key R & D Plan (2017YFB0406301) and Hubei Provincial Innovation Group Project (2019CFA004).
Article link: https://www.sciencedirect.com/science/Article/pii/s1359645421003451